Lithium-ion batteries play a critical role in advancing sustainability efforts. From eco-friendly mobility to clean energy, many sustainability initiatives rely on this technology. This has resulted in a surge in the demand for lithium-ion batteries. Electric vehicles and energy storage systems are the primary applications driving this demand. According to the World Economic Forum, there has been a 50-fold increase in the sale of EV cars between 2012 (200,000 units) and 2022 (over 10 million). (1)

Images source: EV volumes (2) via World Economic Forum (3)
Projections indicate that annual sales may almost double to 17 million units in the next five years. An increase in production is matching the rise in the demand for lithium-ion batteries. However, this trend raises some concerns. Lithium battery production in gigafactories has a scrap rate of 10% to 30% across the various production processes involved, according to Circular Energy Storage. (3) While several innovations are driving down production scrap rates, production waste still accounts for more than 60% of existing battery scrap. Additionally, Lithium batteries have an average life span of 15 years. This means that in just over a decade, millions of end-of-life batteries will be discarded annually.
In addition to these concerns, experts are projecting material shortages in certain battery production materials. A comprehensive solution to mitigate these challenges effectively is recycling, and material recovery.
The importance of Li-Ion battery recycling
Recovery of raw materials: Many raw materials utilized in battery production are finite resources obtained through mining. The increased demand for battery raw materials, resulting from the exponential shift towards sustainable energy and EV production, poses the risk of the rapid depletion of available resources, with experts predicting material shortages in the future.
Considering these figures, additional sources of raw materials may be required to sustainably meet steeply rising demands for certain metals if the current growth rates continue. Recycling end-of-life batteries provides a sustainable source of raw materials to complement the mined materials.
Environmental protection: Li-Ion batteries can severely impact the environment when improperly disposed of. These batteries contain environmentally toxic materials that can leach out and contaminate land, water bodies, and entire ecosystems. Additionally, batteries have the propensity to spontaneously combust and start fires in landfills and dumpsites. A BBC article reported that lithium-ion batteries from electronics were responsible for over 700 fires yearly in waste processing centers across the UK, causing over £158 million worth of damage a year. (4) Furthermore, the incineration of Li-Ion batteries releases toxic gases such as SO2, HCl, HBr, HF, HCN, and NOx, requiring extensive pollution abatement processes and additional safety equipment. These factors make it imperative to dispose of used batteries properly through recycling.
Value recovery: In addition to fulfilling environmental responsibilities, battery recycling also offers considerable financial value. For manufacturers, it subsidizes expenditure on sourcing new raw materials. For independent recyclers, the valuable materials extracted can be sold for profit. In both scenarios, the recovered value typically exceeds the recycling expenses.
The image below shows the material cost composition in USD of EV battery cells by weight. Note that the compositions of different types of Li-ion batteries vary widely. The battery explored is a Chevy Bolt 60 kilowatt-hour (kWh) capacity battery (7). The prices are based on the cost per weight of the materials as of November 2023. Note that the prices of the raw materials do not reflect the actual price of an EV battery as this is influenced by several other factors.

EV battery recycling is a profitable, fast-growing, relatively new market propelled by regulation, environmental responsibilities, high demand, and projected material shortages.
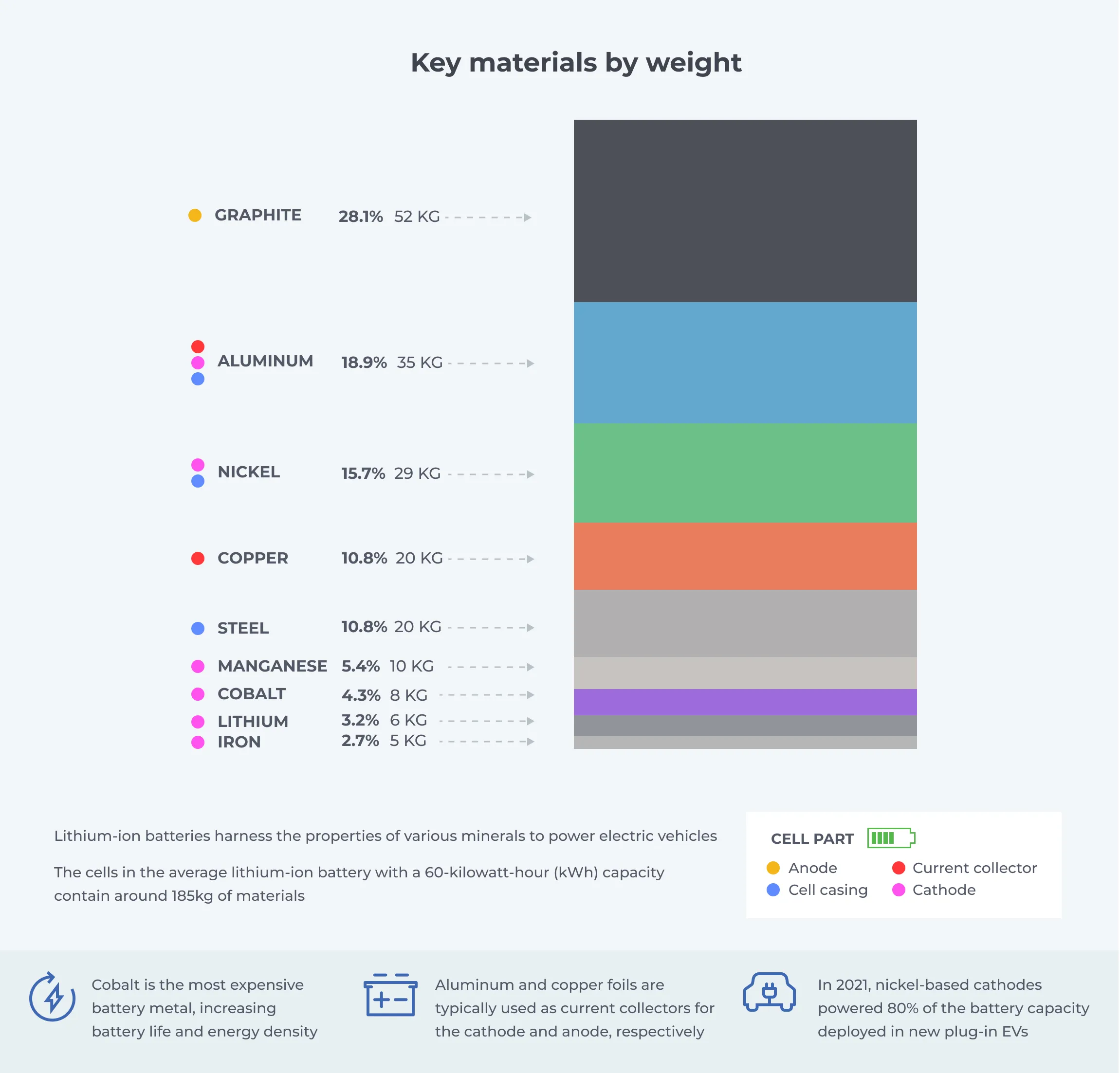
The current state of lithium-ion battery recycling
Production vs. Recycling capacity
Battery production volumes around the world are at an all-time high. These figures are expected to continue rising in the next few years with growing interest and investments in EV and renewable energy production. The European Battery Alliance projects a 12-fold increase in battery production across Europe from 80 GWh in 2022 to about 1000 GWh in 2030. Similar growth rates are expected for North America and Asia. (5)
There has been a corresponding increase in recycling capacity for waste batteries. Recognizing the critical need for battery recycling, an increasing number of battery manufacturers are taking responsibility for waste and end-of-life batteries by creating/investing in in-house battery collection and recycling capabilities to create a circular supply chain. Battery production giants like Volkswagen, Tesla, and Northvolt already have in-house EV battery recycling capacity, while many others have announced plans to develop similar capabilities. Outside of closed-loop supply chains, third-party independent recycling entities play a key role in closing the gap between production and recycling volumes.
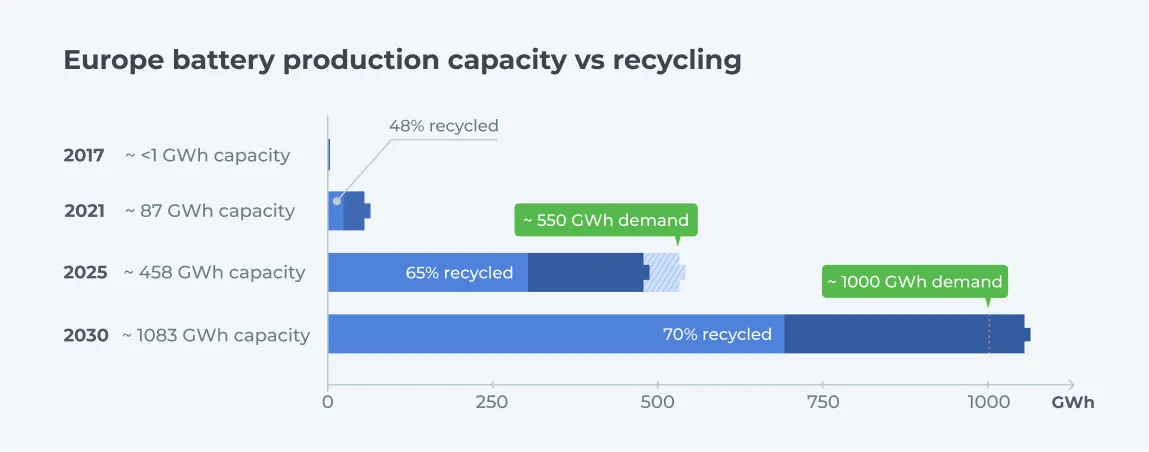
Sources: European Battery Alliance, https://www.transportenvironment.org/wp-content/uploads/2021/08/Battery-brief-1.pdf, https://ec.europa.eu/eurostat/statistics-explained/index.php?title=Waste_statistics_-_recycling_of_batteries_and_accumulators
As shown above, the European recycling capacity is growing rapidly to match or exceed the expected end-of-life explosion over the next 10-15 years. Increased OEM investment toward a circular supply chain encompassing circular battery design, integrated waste collection, and efficient recycling is driving the increase in capacity. Furthermore, stricter, comprehensive government regulations, such as battery passports, and increased involvement from third-party recycling entities are also making a significant impact.
Working principle, composition, and types of lithium-ion batteries
Numerous battery technologies have been developed and used over the years, from lead acid and lithium to Nickel/Cadmium and many others that are still used in countless applications. Today, lithium-ion batteries are the most common technology used for energy storage in electric vehicles, renewable energy, and numerous other applications across various industries. Its power-to-weight ratio, high energy density, long lifespan, and low self-discharge have propelled this technology to the forefront.
A lithium battery typically comprises four major components: a cathode, an anode, an electrolyte through which the ions flow, and a separator - a porous material that separates the cathode and the anode but allows ions to flow through. Batteries also feature current collectors at the electrodes. The cathode and anode are submerged in the electrolyte and are connected in an external circuit via the current collectors.
Lithium ions migrate between the anode and the cathode through the conductive electrolyte, causing electrons to move between electrodes in the external circuit. The movement of electrons provides a current that charges the connected system. During the charging of the battery, electrons from the charger are deposited at the anode. This causes positively charged lithium ions to migrate through the electrolyte from the cathode to the anode, where they gain the deposited electrons.
During discharge, the lithium atoms at the anode gradually lose electrons. These electrons move from the anode to the cathode, generating the current that supplies the connected system in the process. Simultaneously, the lithium atoms revert to positively charged ions (as they have lost electrons) and migrate back to the cathode. When migration is complete, the battery is completely discharged, and the process is repeated by charging the battery. (6)

The anode, cathode, electrolyte, and separators are made from various materials with distinct characteristics that enable Li-Ion batteries to work. These materials, which include both metallic and nonmetallic elements and compounds, are the focus of battery recycling, as most of them are valuable substances that can be recovered and reused.
The anode is typically made from graphite encapsulating a copper current collector. Lithium titanate (Li4Ti5O12) is also a popular anode material for batteries.
The electrolyte comprises a lithium salt dissolved in an organic solvent such as ethylene carbonate, dimethyl carbonate, or diethyl carbonate. Lithium hexafluorophosphate (LiPF6) is the most used in EV vehicles. Lithium tetrafluoroborate and lithium perchlorate are also frequently used.
Current collectors are typically made from aluminum for the cathode and copper for the anode.
Various materials are used as cathodes in EV batteries. The cathode composition is the major distinguishing factor among the types of Li-Ion batteries. It influences the cost, power, lifespan, safety, and battery performance. The types of lithium-ion batteries based on their cathode technologies are as follows:
- Lithium nickel manganese cobalt oxide (NMC): LiNixMnyCozO2
- Lithium iron phosphate (LFP): LiFePo4
- Lithium nickel cobalt aluminum oxide (NCA): Available in different compositions, the most popular being LiNi0,84Co0,12Al0,04O2
- Lithium Manganese Oxide (LMO): LiMn2O4
- Lithium Cobalt Oxide (LCO): LiCoO2
While they all follow the same broadly described working principle, the battery types have varying characteristics. The difference in materials and volume/percentage composition determines each battery type's performance, benefits, and limitations.
The Li-ion Battery Recycling Process
The battery recycling process typically begins with the transportation of the battery scrap to a recycling facility. For production waste, the manufacturer packages the scrap and transports it to the recycling center. On the other hand, EOL batteries are removed from the vehicles at designated extraction and collection points and shipped for recycling.
The actual lithium-ion battery recycling at the recycling facilities typically involves the following steps:
- Inspection and Discharging
- Disassembly
- Size reduction and separation
- Black mass refining

Inspection and Discharging
At the recycling facility, the batteries are inspected for temperature, external damage, leakages, and residual charges. Batteries are designed not to completely discharge during use. Therefore, end-of-life batteries may still retain some electrical charge. The batteries are typically discharged using discharge load units. This can also be done via cryogenic freezing or submersion in an aqueous solution. They are discharged to below 0.5 V to be safe for the subsequent dismantling and crushing phases of recycling.
Disassembly
End-of-life batteries arrive at processing centers as battery packs, not bare cells. Multiple cells make a module, and multiple modules make a pack. After discharge, the battery is dismantled to separate the lithium-ion cells from other parts of the battery. While this is done manually in most recycling centers, autonomous disassembly machines are being developed. The battery casing is first removed, followed by the extraction of the battery management system (BMS), power electronics, and the thermal management system. After that, wires, cables, and connectors are removed and sorted. Depending on the conditions of these components, they may be repurposed as spare parts for new batteries or further processed into raw materials. Finally, the modules are obtained after disassembling the securing holders. The modules may be further disassembled to obtain individual battery cells.
Size reduction and separation
The cells are crushed, pulverized, or shredded into particles. Some facilities skip the dismantling process and shred the battery packs whole after discharging them. The shredded particles are separated via a series of various techniques such as sieving, magnetic separation, air suction, electrostatic separation, and liquid-based sorting. One common technique is to vibrate the particles through a series of sifters and screens that separate the leftover plastic from the mass of particles. Another liquid-based sorting technique is to submerge the particle in a liquid and then recover the light floating plastic from the top and the heavier metal pieces at the bottom.
The metals recovered at this stage typically include copper, aluminum, and steel. These metals, as well as the recovered plastic, are sorted and stored for further processing. After the plastic and metal particles have been removed from the shredded mass, what is left is a black, powdery substance known as black mass. Black mass refining is the last stage of Lithium-ion battery recycling.
Black mass refining
Black mass is an active powder comprising a combination of cathode and anode materials and other components like electrolytes and conductive additives. The black mass is named after its color, which results from the high graphite content from the battery anode. This highly valuable material typically contains a mixture of lithium, nickel, cobalt, manganese, copper, and other recoverable metals, many in their oxide form. The two most common techniques for extracting these metals from black mass are pyrometallurgy and hydrometallurgy. Different recyclers take different approaches to black mass processing.

Pyrometallurgy
Pyrometallurgy involves incinerating the black mass in a smelter to very high temperatures of up to 1,400°C, where the black mass melts. This technique relies on the difference in temperatures and thermodynamic reactions between the constituent elements in black mass and added reducing agents to separate the materials. During this process, the graphite and any remaining electrolyte in the black mass burn off. The nickel, cobalt, and copper content form a mixed metal alloy, while the lithium and manganese form slag that floats to the top of the melt.
Pyrometallurgy is a relatively straightforward process. However, it has several drawbacks. This process consumes large amounts of fuel to achieve the high temperatures required. It also requires sophisticated pollution abatement processes to prevent severe environmental pollution. In addition, pyrometallurgy extracts the metals in forms that need further processing. The elements that form the alloy matte require further processing, such as electrorefining, to separate them. The other elements, lithium, and manganese, form slag that can be difficult and expensive to extract. This may result in lithium losses and low recovery rates.
Hydrometallurgy
Hydrometallurgy is a more common technique of refining black mass as it typically has a lower initial capital outlay. Hydrometallurgy relies on a series of chemical reactions to extract the minerals from black mass. This technique typically involves leaching - dissolving the black mass in an acid to form a solution, followed by purification/extraction techniques such as precipitation, solvent extraction, and ion exchange that selectively recovers the constituent metals from the black mass. Hydrometallurgical techniques can vary significantly based on the choice of chemicals and reagents, extraction methods, the order of extraction, and the form in which the metals are recovered. A sample technique is outlined as follows:
- Leaching and graphite removal: The black mass is dissolved in an acid, such as sulfuric acid (H2SO4), in a process known as leaching. This dissolves the metals, leaving the graphite for filtration.
- Solvent Extraction or Precipitation for Copper: Appropriate reagents are used to extract copper from the solution via solvent extraction (copper sulfate, CuSO4) or precipitation (copper sulfide, Cu2S).
- Precipitation: Quicklime (CaO) is introduced to the solution, causing the precipitation of residual aluminum, iron, and zinc as their hydroxides Al(OH)3, Fe(OH)3, Zn(OH)2, along with calcium sulfate (CaSO4). These are filtered out.
- Solvent Extraction for Nickel, Cobalt, and Manganese: Solvent extraction separates and recovers nickel, cobalt, and manganese as sulfates.
- Lithium Recovery: Sodium carbonate (Na2CO3) is added to the solution, forming crystallized lithium carbonate, and leaving sodium sulfate (NaSO4) behind which needs to be recycled.
It is important to note that hydrometallurgical processes can vary considerably. The provided technique demonstrates a step-by-step recovery of constituent metals in black mass using specific reagents and extraction methods. Variables such as the choice of reagents, recovery techniques, the order of recovery, and the state in which the metals are recovered (sulfates, hydroxides, or carbonates) can vary. Specific procedures like filtration, heating, and pressurization may also differ in practice.
Copper removal from black mass
The removal of copper is a vital step in black mass refining. Copper accounts for 9 - 15% of EV batteries' composition, depending on the battery type, and is used in cathode production. Some of the techniques for copper extraction are as follows.
Hydrometallurgy
As outlined earlier in the article, copper can be extracted from black mass using physical separation and/or hydrometallurgy. In the hydrometallurgical process, the black mass is typically leached using sulfuric acid, and copper is recovered from the solution via precipitation or solvent extraction. This process can be complex and expensive, requiring specialized equipment, advanced expertise, and the use of consumable reagents. Several processing steps are typically required to obtain high-purity, usable copper that can either be sold or used to produce foil-grade copper for reuse in batteries.
Electrowinning
Electrowinning utilizes the principles of electrolysis to extract copper from a black mass leach solution via an electrolytic chemical reaction. This technique requires a negative cathode, a positive anode, and the copper-ion-containing electrolyte.
When an electric current passes from the negatively charged cathode to a positively charged anode through the solution, the electrons reduce the copper ions in the solution to form a solid copper deposition on the cathode. Electrowinning recovers copper from the electrolyte in the form of high-purity cathodes. However, the other dissolved metals will compete with copper to electro-deposit on the cathode as an impurity. This normally requires pre-treatment or post-refining to ensure the production of high-quality copper cathodes.
Conventional electrowinning also has inherent selectivity, operating cost, and safety limitations. Conventional cells have lower mass transfer rates allowing competing ions (impurities) to be electrodeposited, reducing the purity of the copper cathode. As copper concentration decreases below around 20-30g/L, the copper product quality drops, and additional processing steps are required. Another limitation of conventional electrowinning is that open cells generate acid mist, requiring significant air handling and extraction systems to keep safe work environments around the cells.
emew electrowinning
emew electrowinning is an advanced and enhanced form of electrowinning that solves many of the limitations of conventional electrowinning and offers numerous benefits. The electrolyte is circulated rapidly past the anode and cathode at a very high flow rate, significantly enhancing mass transfer and improving recovery efficiency. This also eliminates the residence time of competing ions around the cathode, significantly reducing the possibility of deposition. emew also offers electrolyte management systems to ensure smooth operation and consistently high-quality copper production.

During the copper electrolysis, acid is regenerated in the cell and can be recycled to the copper removal process, thus reducing overall acid consumption and waste generation. Furthermore, emew electrowinning cells are a closed system that overcomes hazardous issues such as acid mist and other noxious gases, making for a much safer working environment. With the emew’s “auto-stripping” of copper cathode tubes from titanium starter sheets (mother blanks), additional stripping equipment is unnecessary, as is the case for conventional electrowinning cells.
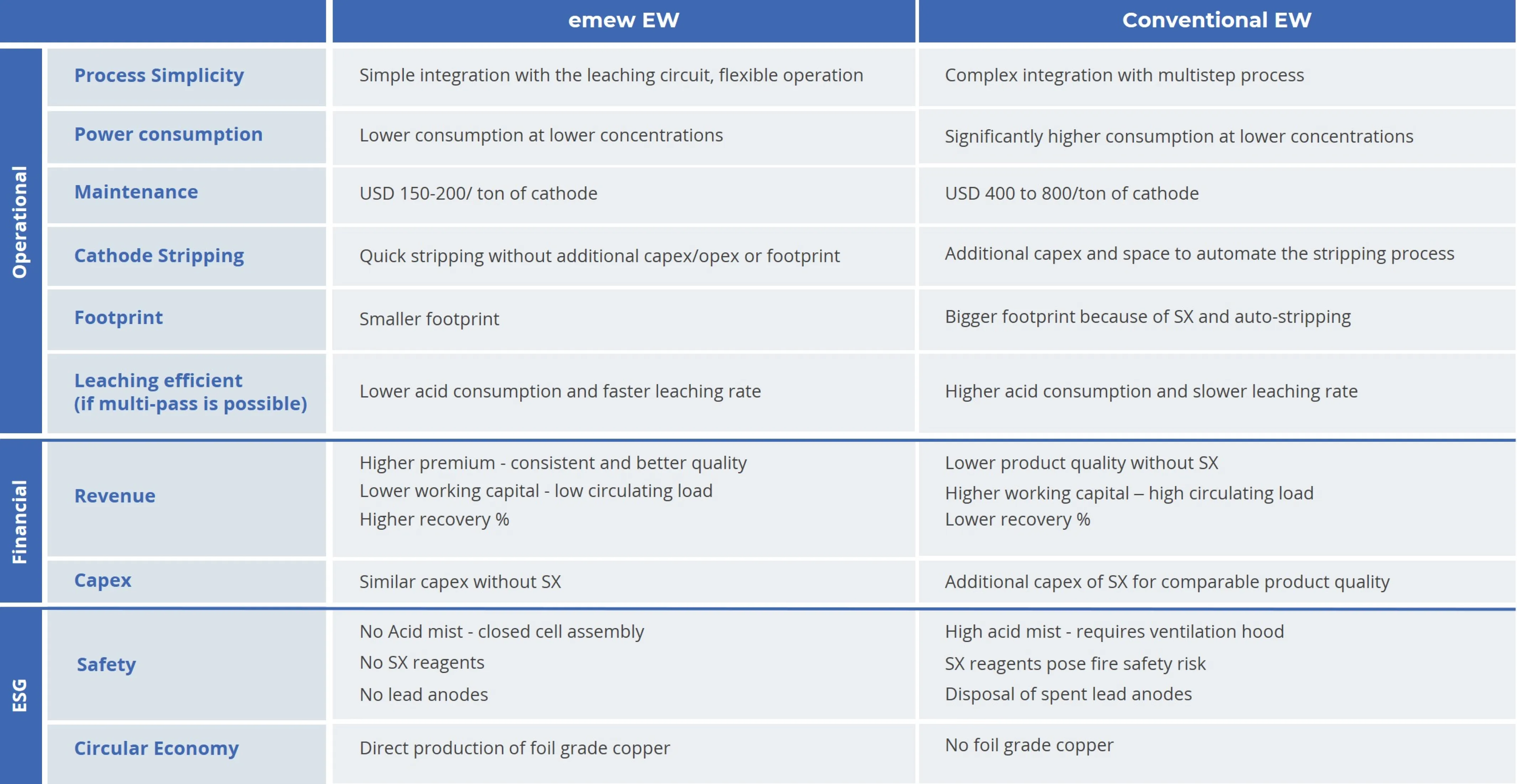
In addition, the emew system recovers copper from a wide range of concentration levels. emew utilizes two technologies to extract copper from Li-Ion battery black mass leachate to very low concentrations. emew Plate removes over 90% of copper from nickel-pregnant leach solutions to below 5 g/L while emew Polish removes the balance enabling 99.9% overall copper recovery to be achieved. The fully decopperized solution is suitable for further processing and extraction of the other constituents of the black mass leachate.
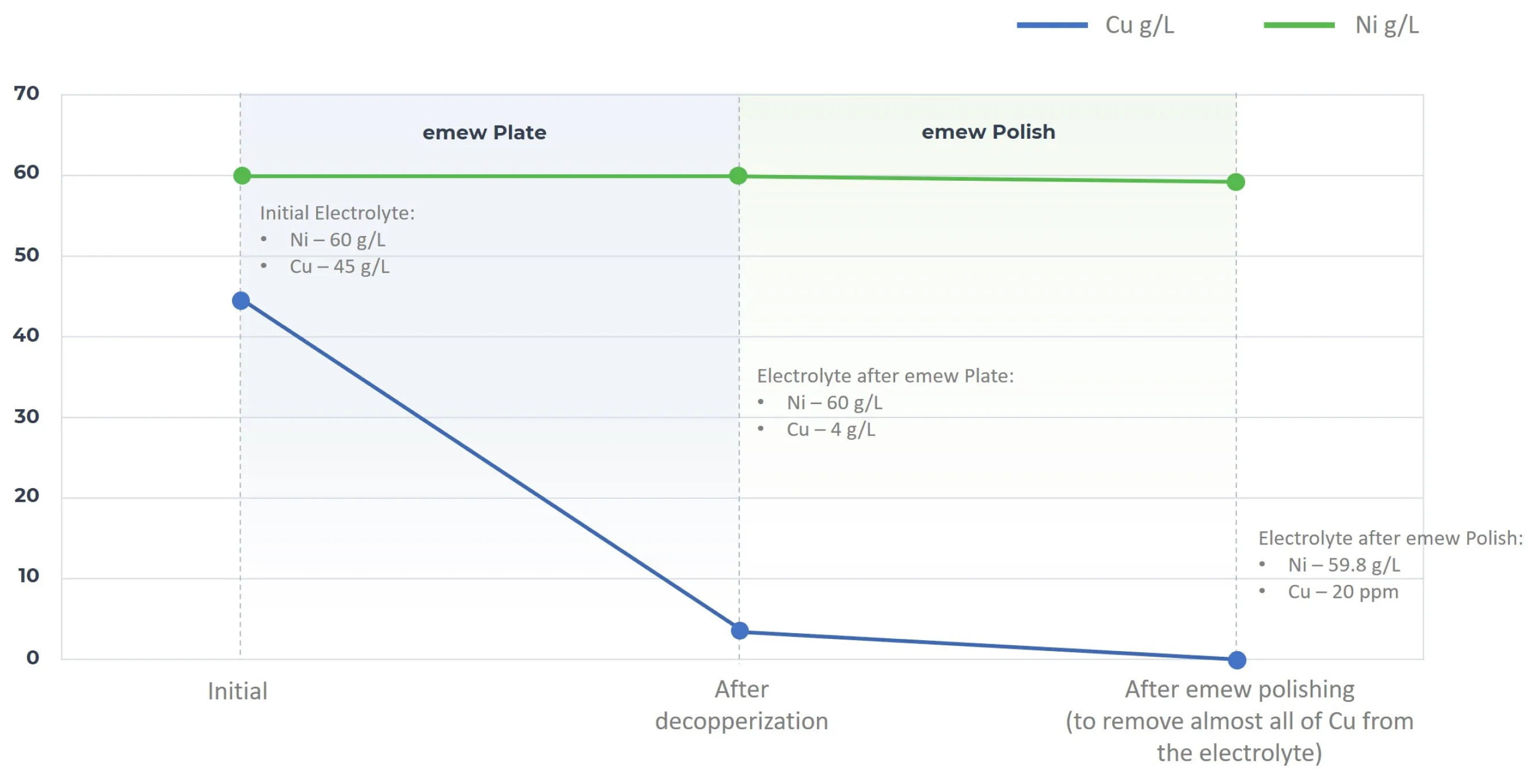
Conclusion
Material recovery from waste EV batteries is not only a vital environmental necessity and a source of raw materials, but it also provides manufacturers and independent recyclers with significant economic value. While many battery technologies are being used and about to reach commercial scale, it is encouraging to see that new Lithium-ion battery recycling technologies are also being developed and deployed at a rapidly growing rate. A combination of product stewardship, government regulations, and economic drivers are pushing the development of LIB recycling technologies into new and exciting developments. In all of these keeping processes safe, simple, sustainable, and flexible to meet future demands and changes is critical to a closed-loop battery economy.
Sources
- https://www.weforum.org/agenda/2023/05/electric-vehicles-ev-sales-growth-2022/
- https://ev-volumes.com
- https://circularenergystorage.com/articles/2022/6/16/the-good-news-about-battery-production-scrap
- https://www.bbc.com/news/science-environment-63809620
- https://www.eba250.com/
- https://www.energy.gov/energysaver/articles/how-lithium-ion-batteries-work
- https://elements.visualcapitalist.com/the-key-minerals-in-an-ev-battery/
- https://www.adlittle.com/sites/default/files/viewpoints/ADL_European_battery_recycling.pdf